Biomechanical and tribological properties of the temporomandibular joint: a narrative review
Introduction
The temporomandibular joint (TMJ) contributes to controlling loads exerted by mandibular movements. Adequate loading in the TMJ promotes tissue remodeling (1). Tissue remodeling is a crucial event for normal functional demands, enhancing homeostasis of the joint. However, excessive and/or abnormal mechanical stress to the TMJ may lead to degradation and abrasion of the joint components (2). Functional overloading and increased joint friction may interact as etiological factors capable of initiating changes in structure of the TMJ (3,4). In particular, fatigue failure and damage of the joint components are linked to loop and sustained compression and shear movements (5-7).
Epidemiologic literatures reported that approximate 25% of the population exhibit some symptoms of TMJ disorders (TMDs) such as joint sound, joint and/or masticatory muscle pain, and limited mouth opening, and one-fifth of those need treatment (8,9). Osteoarthritis in the TMJ (TMJ-OA) is known as the end stage of TMDs, and most of TMJ-OA patients exhibit joint pain as a main symptom. When the joint deterioration starts, TMJ-OA impairs patients’ health, leading to various structural and functional degradation (10).
Taken these considerations, better understanding the mechanical microenvironment in the TMJ is a key step for the development and progress of effective treatment remedy for TMJ-OA. To develop an evidence-based approach to clinical management and treatment for TMJ-OA, we should do fully effort to TMJ biomechanics including biomechanical and tribological properties of the TMJ components, leading to the strategy for joint regeneration and tissue engineering in future (Figure 1). This paper reviews the biomechanical properties of the TMJ components, and the tribological properties of the TMJ lubrication. In addition, the possibility of TMJ computed simulation will be discussed.
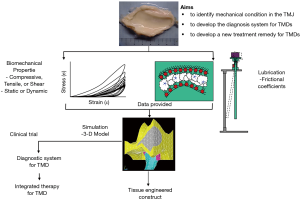
Methods
Search strategy
PubMed, MEDLINE, and Web of Sciences were searched to retrieve relevant articles published from January 2000 to December 2020 using the following keywords: temporomandibular joint, friction, joint lubrication, and tribology.
Study selection
Inclusion criteria were original articles and review articles with a precise definition of the TMJ, published in English, time of publication: January 2000 to December 2020. Relevant publications from the list of references were retrieved and further analyzed to examine whether they matched the inclusion criteria. Data retrieval and extraction were performed by the author. The quality of the retrieved articles was not elucidated.
Part I. Biomechanical properties of the temporomandibular disc and cartilage
Mandibular movements induce various loading in the TMJ, which is divided into static and dynamic loading (see Appendix 1). For instance, clenching, grinding and bruxism result in static loading; talking and chewing include dynamic loading. It is generally accepted that dynamic loading is subject to anabolic effect in the joint components, while static loading is subject to catabolic effect. In addition, there are three loading types: compression, tension, and shear. During mandibular movements, these loading types act together on the articulating surfaces. By joint loading, the condylar and temporal cartilages and the TMJ disc suffer from deformations, which depend on their biomechanical properties. Furthermore, Kim et al. (11) indicated that the subchondral bone is also responsible for bearing static and dynamic loading in humans.
Previously, the elastic behaviors of the TMJ disc and cartilage have been investigated. The tensile moduli mainly depend on the amount and orientation of collagen fibers (6,12,13). Furthermore, elastin fibers in the TMJ disc might interact with collagen fibers, resulting in its sufficient resistance to tension (14,15). The compressive moduli mainly depend on the density of the proteoglycans, especially large chondroitin sulfate (6,13). Exploration of shear behaviors in synovial joints is of great importance, because shear loading is associated with fatigue, damage and degeneration of cartilage (6,16). The shear modulus of the TMJ disc in human is lower than their tensile and compressive moduli, but it increases with age (16), leading to secondary tissue damage.
The average of the elastic modulus in the TMJ disc was 25–30 MPa, while the elastic modulus of the mandibular condylar cartilage was 5–12 MPa (17-19). Recent study indicates both TMJ disc and mandibular condylar cartilage had similar magnitudes of values and behavior under unconfined compression (20). Comparing to the elastic moduli in the other tissues, the average value in the TMJ disc was almost similar to those in the intervertebral disc and knee meniscus, which was smaller than those in tendon and ligament, and larger than those in articular cartilage. Considering the magnitude of TMJ loading during function, the TMJ disc and mandibular condylar cartilage were stiff enough to work as a stress absorber and shock absorber in the TMJ and enable functional joint movements (6,13,21). In summary of static tests, the TMJ disc and mandibular condylar cartilage show a viscoelastic behavior during various movements, and through this behavior, they play essential roles as a stress-absorber and protector for the surrounding tissues.
The dynamic properties of the TMJ disc and articular cartilage are generally increased with increments of loading frequency and strain. For instance, during dynamic compression of the TMJ disc, the maximal stress and the resultant energy dissipation became greater with increases of the indentation amplitude and frequency (22-24). Similarly, the dynamic shear and compressive moduli of the mandibular condylar cartilage were increased nonlinearly with increasing frequency irrespective of the strain amplitude (25-29). Furthermore, it is accepted that the curves with the experimental top and bottom stresses in cyclic loading are close or similar to the theoretical stress-relaxation curves according to the quasi-linear viscoelastic theory (30-32).
Furthermore, dynamic shear behaviors of the TMJ disc and condylar cartilage are anisotropic (8,33-35) (see Appendix 1). Under dynamic shear in the antero-posterior direction, a storage modulus (G’) of about 1.0–1.5 MPa and a loss modulus (G”) of about 0.2–0.3 MPa were found in the TMJ disc (8,32,33), while a storage modulus of 1.5–2.0 MPa and a loss modulus of 0.4–0.5 MPa were in the mandibular condylar cartilage (34,35). The dynamic shear modulus was approximately 3–5 times smaller in the medio-lateral direction than in the antero-posterior direction, which means that the TMJ disc and mandibular condylar cartilage exhibit weak in the masio-lateral shear compared to in the antero-posterior shear.
By a series of studies, we properly understood the biomechanical behaviors of the mandibular condylar cartilage and TMJ disc in both static and dynamic aspects. The mandibular condylar cartilage and TMJ disc contribute to stress reduction and distribution in the TMJ components, mandibular movement promoter and energy dissipation within the joint tissues. Without the energy dissipation due to the disc, TMJ components including mandibular condyle and articular cartilage might fail leading to TMJ-OA (36).
Part II. Tribological properties of the TMJ lubrication
In healthy TMJ, the disc smoothly moves forward and downward during jaw opening without discomfort and pain, and the frictional force between the disc and articular cartilage surfaces has been considered to be negligible because of the presence of healthy synovial fluid. When the synovial fluid degenerates and its viscous property downregulates subsequently, it is likely to remove from the articular surfaces during jaw movements. This means a reduction of joint lubricants from the articular surfaces. Therefore, to study the frictional coefficient in the TMJ is of great importance for evaluating biomechanical microenvironment in the TMJ.
Generally, lubrication modes in synovial joints can be divided into fluid film and boundary lubrication (see Appendix 1). The fluid film lubrication mainly depends on synovial fluid, and the boundary lubrication on joint components such as the TMJ disc and articular cartilages. Immediate after shear and/or compression, the interstitial fluid in articular cartilage has pressure caused by the biphasic tissue structure. Synovial fluid is also thought to be pressurized status between articular surfaces (37). Pressurized interstitial fluid may contribute to bearing compressive force but not resistance to shear force (38).
In healthy joints, the frictional coefficient between articular surfaces has been reported a range of 0.001–0.1 (39-42). For the TMJ, we firstly reported the frictional coefficient of porcine TMJ by using the pendulum-type friction tester (43,44), although Nickel and McLachlan (45) investigated the coefficient of friction between the TMJ disc and acrylic resin plate. The mean frictional coefficient of intact porcine TMJ was 0.0164±0.0020 at the onset of loading with a 50-N (Table 1). The loading time longer, the greater the frictional coefficient. The frictional coefficient of the TMJ exceeded 0.0220±0.0014 after the prolonged loading. With an 80-N load, the frictional coefficient was on average 0.0191±0.0021 at the onset of loading, which was significantly greater than that with a 50-N load. This indicates that longer and/or larger loading to the TMJ induces an increment of the frictional coefficient in intact porcine TMJ, although the maximum values of frictional coefficients in the TMJ are within the normal range in synovial joints.
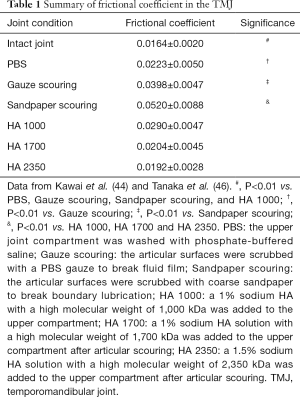
Full table
It is well recognized that an increase of frictional coefficient is one of the major trigger for disc displacement (47). We also evaluated the effects of lubrication breakdown on the frictional coefficient in the porcine TMJ (44,46,48). First, to experimentally reperceive breakdown of fluid lubrication, the articular surfaces of the TMJ disc and cartilage were cleansed with phosphate-buffered saline (PBS) (44). The synovial fluid was expelled from the joint cavity and replaced with PBS. By the breakdown of fluid lubrication, the frictional coefficient of the porcine TMJ was significantly increased to 0.0223±0.0050 (Table 1). Furthermore, to break down the boundary lubrication, the articular cartilage surface was wiped the fluid off with PBS gauze (44). As the result, the coefficient of friction in the porcine TMJ was further increased to 0.0398±0.0047 (Table 1). This indicates that the breakdown of both fluid film and boundary lubrication synergistically affects the coefficient of friction in the TMJ. In addition, to elucidate the effect of incongruent articular surface on the frictional constant in the TMJ, the amorphous layer of the articular cartilage was disrupted by scouring with sandpaper, resulting in producing the OA-lesion. After scouring with sandpaper, the frictional coefficient of the porcine TMJ tremendously increased to 0.0520±0.0088 (Table 1). These findings suggest that articular congruency also affects the frictional coefficient in the porcine TMJ.
Hyaluronic acid (HA), non-sulfate glycosaminoglycan, is one of the principal components of synovial fluid playing a crucial role in the rheological biomechanics of synovial joints (49). Although the concentration and molecular weight of HA are different with age, synovial fluid in healthy joints contains high molecular weight HA, while OA joints include much amount of low molecular weight HA, leading to the inflammatory condition. Synovial viscosity is dependent on both the concentration of HA and its molecular weight. In OA-joints with much amount of low molecular weight HA, the synovial fluid has a reduced viscosity. For this reason, it has been used for around 50 years to treat knee OA in humans. Many studies reported and confirmed the meaningful benefit of HA supplementation in OA treatment (50,51). For TMJ-OA, exogenous viscosupplementation has been recognized to relieve joint pain and increase maximal jaw opening without pain in patients with TMJ-OA (52). Especially, HA with high molecular weight may be the better candidate to improve masticatory system in TMJ-OA. Therefore, the role of HA in TMJ lubrication was recognized clearly by the measurements of the frictional coefficients in the TMJ after the application of high molecular weight HA (44,46).
After applying additive high molecular weight HA to incongruent joint like TMJ-OA joint, the coefficient of friction was decreased significantly by 43-56%, although the coefficient of friction did not recover to the level of the intact joints even if application of high molecular weight HA (44,46). Taken together, these studies confirmed the beneficial effect of high molecular weight HA in TMJ-OA.
Regarding the mechanism of TMJ lubrication, Nitzan (47) strongly suggested that surface active phospholipids (SAPLs) enhance boundary lubrication in the TMJ and play a role as protector of intracapsular articular surfaces. SAPLs are polar lipids which connect with the articular surface via their polar ends, thus orientating their non-polar moieties outward. The non-polar moieties provide a hydrophobic function, by which the articular surface has a relatively lower surface energy, resulting in less ability of friction. HA with high molecular weight locates between articular surfaces and protects the SAPLs from direct invasion by phospholipase A2 (PLA2). PLA2 is secreted from synoviocytes, chondrocytes, and osteoblasts into synovial fluid, and its activity induces the lysis of the SAPLs. To confirm this mechanism of TMJ lubrication, the effect of SAPLs on the frictional coefficient in the TMJ was examined (53). After treatment with bovine pancreas secreted PLA2, the SAPLs on the articular surfaces disappeared. Furthermore, the frictional coefficient of the sPLA2-treated mandibular condyle was significantly higher than that of intact joint (53). We also measured the frictional coefficient in porcine mandibular condyle after digestion with hyaluronidase to examine the role of HA in synovial fluid in joint lubrication (54). As the result, the coefficient of friction in the porcine TMJ significantly increased by 35% after treatment with hyaluronidase.
A mucinous glycoprotein called proteoglycan 4 (PRG4), also known as lubricin (55,56), covers the articular surfaces and functions synergistically with HA (57,58). This enables to form non-contiguous nanofilm, resulting in its lubricating and antiadhesive properties when connecting with the articular surface (59). Apart from the lubrication function, several studies demonstrated that PRG4 plays a crucial role in synovial cell proliferation and adhesion (57,60). PRG4 can mediate the proliferation of synovial cells for maintenance of cartilage surfaces (60). PRG4 knockout mice show synovial hyperplasia, deterioration of articular cartilages in the TMJ with an enhancement of chondrocyte proliferation and their redistribution in clusters with loss of superficial zone chondrocytes (60). Furthermore, PRG4 concentrations were significantly decreased in the synovial fluid of TMJ-OA (61). These findings indicate that PRG4 exerts essential direct and indirect roles to preserve TMJ structural and cellular integrity (62). Intraarticular supplementation with PRG4 might be an effective remedy for TMJ-OA with loss of joint lubrication (57).
In summary, the TMJ lubrication system is a key mediator for mandibular dynamics. To understand the development and breakdown mechanisms of the TMJ lubrication is indispensable to a novel new treatment remedy for TMJ-OA.
Final remarks
The biomechanical models of human masticatory system are powerful tools for evaluating the biomechanical microenvironment in the TMJ. Previously many researchers have attempted to develop two-dimensional or three-dimensional TMJ models for simulation and animation of the TMJ motion and stress analysis in the TMJ during masticatory function without invasive approach (22,55-57,63-66). With a revolution in computer science, the models have been in reality simulations including four-dimensional simulation. Furthermore, the biomechanical and tribological information about the TMJ components as described above enables to approach complete real model as much as possible (Figure 2). Obviously, the numerous assumptions underlying these FE models of the human TMJ should be addressed when interpreting its predictions even if obtaining numerous information (67). Moreover, the results obtained from FE analysis cannot be immediately adopted to clinical practice without further consideration. Nevertheless, it must be emphasized that the strength of this method is that it may enable us to perform intervention study.
As a conclusion, biomechanical model of the human TMJ and its application to stress analysis during mandibular movements are absolutely not perfect. Except for the model analysis, however, we have no way to examine the biomechanical microenvironment in the TMJ by no or less invasive procedure. In contrast, better understanding of biomechanical environment within the TMJ is absolutely necessary for the diagnosis and prognosis of treatment of masticatory dysfunction. Future studies with much efforts are required to measure and visualize the biomechanical microenvironment within the TMJ during mandibular movements in clinical aspect.
Acknowledgments
Funding: None.
Footnote
Conflicts of Interest: The author has completed the ICMJE uniform disclosure form (available at https://fomm.amegroups.org/article/view/10.21037/fomm-20-83/coif). The author serves as an unpaid editorial board member of Frontiers of Oral and Maxillofacial Medicine from May 2020 to Apr 2022. The author has no other conflicts of interest to declare.
Ethical Statement: The author is accountable for all aspects of the work in ensuring that questions related to the accuracy or integrity of any part of the work are appropriately investigated and resolved.
Open Access Statement: This is an Open Access article distributed in accordance with the Creative Commons Attribution-NonCommercial-NoDerivs 4.0 International License (CC BY-NC-ND 4.0), which permits the non-commercial replication and distribution of the article with the strict proviso that no changes or edits are made and the original work is properly cited (including links to both the formal publication through the relevant DOI and the license). See: https://creativecommons.org/licenses/by-nc-nd/4.0/.
References
- Stegenga B, DeBont LGM, Boering G. Osteoarthrosis as the cause of craniomandibular pain and dysfunction: a unifying concept. J Oral Maxillofac Surg 1989;47:249-56. [Crossref] [PubMed]
- Arnett GW, Milam SB, Gottesman L. Progressive mandibular retrusion - idiopathic condylar resorption. Part I. Am J Orthod Dentofacial Orthop 1996;110:8-15. [Crossref] [PubMed]
- Milam SB, Schmitz JP. Molecular biology of temporomandibular joint disorders: proposed mechanisms of disease. J Oral Maxillofac Surg 1995;53:1448-54. [Crossref] [PubMed]
- Milam SB, Zardeneta G, Schmitz JP. Oxidative stress and degenerative temporomandibular joint disease: a proposed hypothesis. J Oral Maxillofac Surg 1998;56:214-23. [Crossref] [PubMed]
- Tanaka E, Hanaoka K, van Eijden T, et al. Dynamic shear properties of the temporomandibular joint disc. J Dent Res 2003;82:228-31. [Crossref] [PubMed]
- Tanaka E, van Eijden T. Biomechanical behavior of the temporomandibular joint disc. Crit Rev Oral Biol Med 2003;14:138-50. [Crossref] [PubMed]
- Iatridis JC, ap Gwynn I. Mechanisms for mechanical damage in the intervertebral disc annulus fibrosus. J Biomech 2004;37:1165-75. [Crossref] [PubMed]
- Carlsson GE. Epidemiology and treatment need for temporomandibular disorders. J Orofac Pain 1999;13:232-7. [PubMed]
- Carlsson GE, LeResche L. Epidemiology of temporomandibular disorders. In: Sessle BJ, Bryant PS, Dionne RA. editors. Temporomandibular disorders and related pain conditions. Seattle: Seattle Press, 1995.
- Zarb GA, Carlsson GE. Temporomandibular disorders: osteoarthritis. J Orofac Pain 1999;13:295-306. [PubMed]
- Kim DG, Haghighi A, Kwon HJ, et al. Sex dependent mechanical properties of the human mandibular condyle. J Mech Behav Biomed Mater 2017;71:184-91. [Crossref] [PubMed]
- Detamore MS, Athanasiou KA. Structure and function of the temporomandibular joint disc: implications for tissue engineering. J Oral Maxillofac Surg 2003;61:494-506. [Crossref] [PubMed]
- Kuroda S, Tanimoto K, Izawa T, et al. Biomechanical and biochemical characteristics of the mandibular condylar cartilage. Osteoarthritis Cartilage 2009;17:1408-15. [Crossref] [PubMed]
- Fazaeli S, Ghazanfari S, Mirahmadi F, et al. The dynamic mechanical viscoelastic properties of the temporomandibular joint disc: The role of collagen and elastin fibers from a perspective of polymer dynamics. J Mech Behav Biomed Mater 2019;100:103406. [Crossref] [PubMed]
- Fazaeli S, Mirahmadi F, Everts V, et al. Alteration of structural and mechanical properties of the temporomandibular joint disc following elastase digestion. J Biomed Mater Res B Appl Biomater 2020;108:3228-40. [Crossref] [PubMed]
- Lai WFT, Bowley J, Burch JG. Evaluation of shear stress of the human temporo- mandibular joint disc. J Orofac Pain 1998;12:153-9. [PubMed]
- Kang H, Bao G, Dong Y, et al. Tensile mechanics of mandibular condylar cartilage. Hua Xi Kou Qiang Yi Xue Za Zhi 2000;18:85-7. [PubMed]
- Kuboki T, Azuma Y, Orsini MG, et al. The effect of occlusal appliances and clenching on the temporomandibular joint space. J Orofac Pain 1997;11:67-77. [PubMed]
- Singh M, Detamore MS. Tensile properties of the mandibular condylar cartilage. J Biomech Eng 2008;130:011009. [Crossref] [PubMed]
- Chin A, Almarza A. Regional dependence in biphasic transversely isotropic parameters in the porcine temporomandibular joint disc and mandibular condylar cartilage. J Biomech Eng 2020;142:081010. [Crossref] [PubMed]
- van Eijden TM. Biomechanics of the mandible. Crit Rev Oral Biol Med 2000;11:123-36. [Crossref] [PubMed]
- Beek M, Koolstra JH, van Ruijven LJ, et al. Three-dimensional finite element analysis of the human temporomandibular joint disc. J Biomech 2000;33:307-16. [Crossref] [PubMed]
- Fernández P, Lamela MJ, Ramos A, et al. The region-dependent dynamic properties of porcine temporomandibular joint disc under unconfined compression. J Biomech 2013;46:845-8. [Crossref] [PubMed]
- Tanaka E, Kikuzaki M, Hanaoka K, et al. Dynamic compressive property of porcine temporomandibular joint disc. Eur J Oral Sci 2003;111:434-9. [Crossref] [PubMed]
- Tanaka E, Yamano E, Dalla-Bona DA, et al. Dynamic compressive properties of the mandibular condylar cartilage. J Dent Res 2006;85:571-5. [Crossref] [PubMed]
- Tanaka E, Detamore MS, Mercuri LG. Degenerative disorders of the temporomandibular joint: etiology, diagnosis, and treatment. J Dent Res 2008;87:296-307. [Crossref] [PubMed]
- Tanaka E, Pelayo F, Kim N, et al. Stress relaxation behaviors of articular cartilages in porcine temporomandibular joint. J Biomech 2014;47:1582-7. [Crossref] [PubMed]
- Lamela MJ, Pelayo F, Ramos A, et al. Dynamic compressive properties of articular cartilages in the porcine temporomandibular joint. J Mech Behav Biomed Mater 2013;23:62-70. [Crossref] [PubMed]
- Wu Y, Kuo J, Wright GJ, et al. Viscoelastic shear properties of porcine temporomandibular joint disc. Orthod Craniofac Res 2015;18:156-63. [Crossref] [PubMed]
- Woo SLY, Mow VC, Lai WM. Biomechanical properties of articular cartilage. In: Skalak R, Chen S. editors. Handbook of bioengineering. New York, McGraw-Hill Book Co., 1988:1-44.
- Koolstra JH, Tanaka E, van Eijden TMGJ. Viscoelastic material model for the temporo- mandibular joint disc derived from dynamic shear tests or strain-relaxation tests. J Biomech 2007;40:2330-4. [Crossref] [PubMed]
- Barrientos E, Pelayo F, Tanaka E, et al. Viscoelastic properties of the central region of porcine temporomandibular joint disc in shear stress-relaxation. J Biomech 2019;93:126-31. [Crossref] [PubMed]
- Tanaka E, Kawai N, Hanaoka K, et al. Shear properties of the temporomandibular joint disc in relation to compressive and shear strain. J Dent Res 2004;83:476-9. [Crossref] [PubMed]
- Tanaka E, Iwabuchi Y, Rego EB, et al. Dynamic shear behavior of mandibular condylar cartilage is dependent on testing direction. J Biomech 2008;41:1119-23. [Crossref] [PubMed]
- Tanaka E, Rego EB, Iwabuchi Y, et al. Biomechanical response of condylar cartilage-on-bone to dynamic shear. J Biomed Mater Res A 2008;85:127-32. [Crossref] [PubMed]
- Guerrero Cota JM, Leale DM, Arzi B, et al. Regional and disease-related differences in properties of the equine temporomandibular joint disc. J Biomech 2019;82:54-61. [Crossref] [PubMed]
- Schmidt TA, Sah RL. Effect of synovial fluid on boundary lubrication of articular cartilage. Osteoarthritis Cartilage 2007;15:35-47. [Crossref] [PubMed]
- Ateshian GA, Hung CT. Patellofemoral joint biomechanics and tissue engineering. Clin Orthop Relat Res 2005;81-90. [Crossref] [PubMed]
- Forster H, Fisher J. The influence of continuous sliding and subsequent surface wear on the friction of articular cartilage. Proc Inst Mech Eng H 1999;213:329-45. [Crossref] [PubMed]
- Linn FC. Lubrication of animal joints. I. The arthrotripsometer. J Bone Joint Surg Am 1967;49:1079-98. [Crossref] [PubMed]
- Mabuchi K, Tsukamoto Y, Obara T, et al. The effect of additive hyaluronic acid on animal joints with experimentally reduced lubricating ability. J Biomed Mater Res. 1994;28:865-70. [Crossref] [PubMed]
- Mabuchi K, Obara T, Ikegami K, et al. Molecular weight independence of the effect of additive hyaluronic acid on the lubricating characteristics in synovial joints with experimental deterioration. Clin Biomech (Bristol, Avon) 1999;14:352-6. [Crossref] [PubMed]
- Tanaka E, Kawai N, Tanaka M, et al. The Frictional coefficient of the temporomandibular joint and its dependency on the magnitude and duration of joint loading. J Dent Res 2004;83:404-7. [Crossref] [PubMed]
- Kawai N, Tanaka E, Takata T, et al. Influence of additive hyaluronic acid on the lubricating ability in the temporomandibular joint. J Biomed Mater Res A 2004;70:149-53. [Crossref] [PubMed]
- Nickel JC, McLachlan KR. In vitro measurement of the frictional properties of the temporomandibular joint disc. Arch Oral Biol 1994;39:323-31. [Crossref] [PubMed]
- Tanaka E, Iwabe T, Dalla-Bona DA, et al. The effect of experimental cartilage damage and impairment and restoration of synovial lubrication on friction in the temporomandibular joint. J Orofac Pain 2005;19:331-6. [PubMed]
- Nitzan DW. The process of lubrication impairment and its involvement in temporomandibular joint disc displacement: a theoretical concept. J Oral Maxillofac Surg 2001;59:36-45. [Crossref] [PubMed]
- Tanaka E, Hirose M, Koolstra JH, et al. Modeling of the effect of friction in the temporomandibular joint on displacement of its disc during prolonged clenching. J Oral Maxillofac Surg 2008;66:462-8. [Crossref] [PubMed]
- Liu Z, Lin W, Fan Y, et al. Effects of Hyaluronan molecular weight on the lubrication of cartilage-emulating boundary layers. Biomacromolecules 2020;21:4345-54. [Crossref] [PubMed]
- Maheu E, Bannuru RR, Herrero-Beaumont G, et al. Why we should definitely include intra-articular hyaluronic acid as a therapeutic option in the management of knee osteoarthritis: Results of an extensive critical literature review. Semin Arthritis Rheum 2019;48:563-72. [Crossref] [PubMed]
- Jones IA, Togashi R, Wilson ML, et al. Intra-articular treatment options for knee osteoarthritis. Nat Rev Rheumatol 2019;15:77-90. [Crossref] [PubMed]
- Liu Y, Wu J, Fei W, et al. Is there a difference in intra-articular injections of corticosteroids, hyaluronate, or placebo for temporomandibular osteoarthritis? J Oral Maxillofac Surg 2018;76:504-14. [Crossref] [PubMed]
- Tanimoto K, Kamiya T, Tanne Y, et al. Superficial zone protein affects boundary lubrication on the surface of mandibular condylar cartilage. Cell Tissue Res 2011;344:333-40. [Crossref] [PubMed]
- Asakawa-Tanne Y, Su S, Kunimatsu R, et al. Effects of enzymatic degradation after loading in temporomandibular joint. J Dent Res 2015;94:337-43. [Crossref] [PubMed]
- Donzelli PS, Gallo LM, Spilker RL, et al. Biphasic finite element simulation of the TMJ disc from in vivo kinematic and geometric measurements. J Biomech 2004;37:1787-91. [Crossref] [PubMed]
- Pérez del Palomar A, Doblaré M. Anterior displacement of the TMJ disk: repositioning of the disk using a Mitek system. A 3D finite element study. J Biomech Eng 2006;128:663-73. [Crossref] [PubMed]
- Koolstra JH, van Eijden TM. Combined finite-element and rigid-body analysis of human jaw joint dynamics. J Biomech 2005;38:2431-9. [Crossref] [PubMed]
- Teeple E, Elsaid KA, Jay GD, et al. Effects of supplemental intra-articular lubricin and hyaluronic acid on the progression of posttraumatic arthritis in the anterior cruciate ligament-deficient rat knee. Am J Sports Med 2011;39:164-72. [Crossref] [PubMed]
- Schmidt TA, Gastelum NS, Nguyen QT, et al. Boundary lubrication of articular cartilage: role of synovial fluid constituents. Arthritis Rheum 2007;56:882-91. [Crossref] [PubMed]
- Rhee DK, Marcelino J, Baker M, et al. The secreted glycoprotein lubricin protects cartilage surfaces and inhibits synovial cell overgrowth. J Clin Invest 2005;115:622-31. [Crossref] [PubMed]
- Hill A, Duran J, Purcell P. Lubricin protects the temporomandibular joint surfaces from degeneration. PLoS One 2014;9:e106497. [Crossref] [PubMed]
- Wei L, Xiong H, Li B, et al. Boundary-lubricating ability and lubricin in synovial fluid of patients with temporomandibular joint disorders. J Oral Maxillofac Surg 2010;68:2478-83. [Crossref] [PubMed]
- Koyama E, Saunders C, Salhab I, et al. Lubricin is required for the structural integrity and post-natal maintenance of TMJ. J Dent Res 2014;93:663-70. [Crossref] [PubMed]
- Abe S, Kawano F, Kohge K, et al. Stress analysis in human temporomandibular joint affected by anterior disc displacement during prolonged clenching. J Oral Rehabil 2013;40:239-46. [Crossref] [PubMed]
- Barrientos E, Pelayo F, Tanaka E, et al. Effects of loading direction in prolonged clenching on stress distribution in the temporomandibular joint. J Mech Behav Biomed Mater 2020;112:104029. [Crossref] [PubMed]
- Hattori-Hara E, Mitsui SN, Mori H, et al. The influence of unilateral disc displacement on stress in the contralateral joint with a normally positioned disc in a human temporomandibular joint: an analytic approach using the finite element method. J Craniomaxillofac Surg 2014;42:2018-24. [Crossref] [PubMed]
- Hirose M, Tanaka E, Tanaka M, et al. Three-dimensional finite-element model of the human temporomandibular joint disc during prolonged clenching. Eur J Oral Sci 2006;114:441-8. [Crossref] [PubMed]
Cite this article as: Tanaka E. Biomechanical and tribological properties of the temporomandibular joint: a narrative review. Front Oral Maxillofac Med 2021;3:15.