Osseointegration—the biological reality of successful dental implant therapy: a narrative review
Introduction
The modern era of dental implant therapy, however, has a foundation established in the search for a solution to complete edentulism. The technical challenges were focused on how to retain and stabilize complete dentures and as such earliest studies involved the use of subperiosteal implants and transmandibular implants as ways to stabilize and retain dentures (1). Unfortunately, these clinical approaches met with varied success at best and were associated with chronic inflammation and infection leading to bone loss, implant loss and dissatisfaction. In the late 1950s through the early 1980s, another concept emerged and that was the use of metallic root form implants anchored in bone to support a fixed prostheses. The pioneering efforts of Dr. P.I. Branemark were based on fundamental studies of vascularization in bone marrow that used metallic chambers to view this process. These chambers were found to be essentially irreversibly fixed to bone. Dr. Branemark quietly developed and documented commercially pure Titanium ‘fixtures’ and their placement into the parasymphyseal mandible to support fixed dentures. Early publications established the potential of this approach based on clinical cohort studies displaying remarkable 10-year ‘fixture’ and prosthesis survival (2).
The term osseointegration was subsequently defined by Branemark as ““a direct structural and functional connection between ordered, living bone, and the surface of a load carrying implant” (3). This general understanding of the direct interface was appreciated by information obtained from histological observations. The development of ground section histology (4) and advances in Transmission Electron Microscopy (5) permitted the identification of bone opposing the titanium implant surface. In a similar manner, Dr. Schroeder observed the growth of bone into a titanium plasma sprayed implant surface in the absence of ‘formation of a soft tissue bed’ noting that the implant was anchored to bone (6). Prior to the established definition of osseointegration, he suggested that bone was “ankylotically” related to the implant surface. Albrektsson and co-workers optimistically concluded in 1981 that “the technique of osseointegration is a reliable type of cement-free bone anchorage for permanent prosthetic tissue substitutes” (7). Thus, osseointegration had been established as a reproducible biologic outcome associated with the fixation of a metallic root-form or screw-shaped implant in alveolar bone. This article aims to provide an overview of factors affecting dental implant osseointegration. We present the following article in accordance with the Narrative Review reporting checklist (available at https://fomm.amegroups.com/article/view/10.21037/fomm-21-77/rc).
Methods
The relevant literature was identified and consulted through PubMed/Medline.
The foundation for clinical dental implant success
As indicated above, a central factor in the acceptance of osseointegration as a clinically successful therapy or tooth replacement was the early studies in Sweden that produced relatively large cohort studies [e.g., 1,000 subjects (8)] that demonstrated high success with limited biological or clinical morbidity. Such early study outcomes were predicated on strict clinical protocols that underscored key biological principles for attaining osseointegration. The key clinical guidelines included (I) step-wise drilling to limit thermal stress to cells and tissues, (II) achieving primary implant stability, and (III) isolation from the oral environment by adhering to submerged (undisturbed) implant placement for 3–6 months (9). The clinical challenge to reproducibly achieving osseointegration was defined as multifactorial (Table 1).
Table 1
Implant material biocompatibility |
Topographical features of the implant surface |
Bone health (non-infected) and bone quality/quantity |
Surgical technique |
Undisturbed healing phase |
Prosthesis effects (loading, hygiene, esthetics). |
*, adapted from reference (10).
Each of the enumerated clinical factors were, presumably, thought to influence the biological process of bone formation at the titanium dental implant surface. With the evolution of dental implant therapy, the warnings implicit to this list of factors affecting osseointegration remain with us in clinical management of our implant patients. The one exception is the undisturbed (submucosal or two-stage) healing phase; one-stage implant placement under conditions where other factors are not limiting has been proven to provide equal osseointegration success as submucosal or two-stage healing. A two-stage approach may be advantageous when there is sub-optimal primary stability or where excessive forces may be transmitted through the exposed healing abutments (11). How each of these clinical factors affects the biological processes involved in osseointegration was not well characterized or possibly mis-characterized when clinical success was realized. Over the past three to four decades the explosion of cell and molecular biological knowledge has enabled important new insights into the biological processes that direct the formation of the bone-to-implant interface essential to osseointegration success.
Osseointegration observed
As stated above, osseointegration was defined histologically and largely at the light microscopic level to represent the direct contact of formed bone with the implant surface. How this direct bone-to-implant interface forms remains incompletely defined. The concept that the implant surface directly promoted bone formation is valid; both animal and cell culture studies demonstrate that bone and bone-derived cells form bone or bone-like tissue on experimental implant surfaces. With the emergence of osteoblast cell culture studies, the effect of the implant surface bulk chemical composition, surface energy, sterilization effects, were quickly investigated (Table 2). Together, these studied demonstrated that changes in the surface character of the implant could influence cell behavior (12-14). It was further observed that the process of cellular attachment by integrin receptors and signaling through focal contacts was influenced by the nature of the implant surface (14). Implied was that the implant surface itself could be modified to enhance the formation at the bone to implant surface.
Table 2
Bulk chemical composition (e.g., titanium, zirconia, hydroxyapatite) |
Surface energy (wettability) |
Surface topography (microscale, nanoscale) |
Surface modification (protein, ion, drug) |
In the 1960’s and 1970’s when early development of osseointegration was ongoing, scientific knowledge of mesenchymal stem cells (MSCs), immunology, genetics, and genomics was in its infancy or not existent. New basic knowledge emerged and has been applied to the study of osseointegration. Central to this has been the understanding of the MSC’s role in bone formation (15). Applied to osseointegration, the MSC’s function in formation of the bone-to-implant interface has been widely explored. These osteoprogenitor cells are found in multiple tissues including bone and bone marrow. Upon injury, the MSC is recruited to the site of injury (here the implant/wound interface) and contributes to bone regeneration (16). At bone fracture sites, MSCs are recruited quickly to the site and begin proliferation at about 3 days following injury (17). This is promoted by inflammatory responses to injury and the release of growth factors, cytokines and chemokines (considered below).
Important observations have been made regarding osseointegration at the earliest stages of wound healing. The formation of the fibrin blood clot creates a scaffold upon which early cellular events occur. The platelets integral to the fibrin clot are rich sources of growth factors and contribute to osseointegration. Structurally, the fibrin scaffold is necessary for cellular movement from adjacent sites onto the implant surface. This process underscores the concept “contact osteogenesis” as described by Davies (18) where new bone formation occurs on the implant surface and not only toward the surface from adjacent sites of neo-osteogenesis.
The population of the implant surface with osteoprogenitors is followed by their proliferation and subsequent differentiation to osteoblastic cells that form a mineralizing matrix (Figure 1). Osteoblastic differentiation is now well defined (19) and multiple studies have demonstrated that implant adherent osteoprogenitors readily form bone (20,21). This process recapitulates in many ways the process of woven bone formation that begins with the recruitment of MSs and the signaling of osteoinduction. Multiple signals are involved in osteoinduction, however, two molecular ‘switches’ are now defined to be essential for the osteoblast formation. They are transcription factors that regulate osteoblast-specific gene expression and are known as Runx2 and Osterix (SP7). If either of these genes are deleted, osteoblast formation and bone formation is not observed in development (22,23). Thus, the activity of these osteoinductive factors drive bone formation and their expression is observed in implant adherent cells. Other major mediators of osteogenesis include bone morphogenetic protein (BMP) signaling pathway, Wingless-related integration site (Wnt) signaling pathway, and parathyroid hormone (PTH) signaling pathway among others. In addition, adhesive extracellular matrix proteins elaborated at the implant surface also influence osteogenesis (24). All appear to be active in the process of osseointegration.
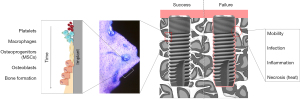
In osteoprogenitor cells on the implant surface, the activity of Runx2 and Osterix is increased in the early phases of osseointegration and direct bone formation at the implant surface (25,26). The early increase in Runx2 and Osterix was affirmed in studies of experimental implants retrieved from humans (27). BMPs are primary inducers of osteoblastic differentiation and they are expressed in implant adherent cells; enhanced surface topography is associated with increased BMP expression and related osteoinduction. BMP expression by implant adherent cells has been further demonstrated using experimentally retrieved implants from humans (28). There are several Wnt ligands that are active in the process of osseointegration and they ultimately activate another transcription factor, β-catenin. Enhanced micron/nanoscale surface topography increases the expression of Wnt proteins that signal the activation of β-catenin to promote osteoblast differentiation and bone formation among implant adherent osteoprogenitor cells. For example, Wnt3A appears to activate local cells contributing to osseointegration (29) and Wnt5a and Wnt11 also play contributing roles (30). Eventually, bone formation ceases in a controlled process. One protein produced by osteocytes that inhibits osteogenesis is Sclerostin. Sclerostin is expressed by implant adherent cells and is increased in the absence of primary stability (31). Antibodies to Sclerostin are used therapeutically to block its damping effect on osteogenesis thereby enhancing bone formation. This has been explored at endosseous implants with positive results (32). Cell directed osteogenesis as the foundation of osseointegration implies that that disruption of the local, systemic or clinical (technical) factor which interferes with osteoprogenitor cell function can interfere with osseointegration. Conversely, it is possible to direct osteoprogenitors to enhance bone formation with the goal of improving osseointegration.
Osseointegration improved
Current titanium dental implants currently possess enhanced surface topography of one-type or another. Implants with surfaces of S(a) values approximating 1.5–2.0 mm may offer increased bone-to-implant contact compared with machined implant surfaces. These surfaces are created in different ways commonly grit-blasting, acid etching or anodizing. Further modification has been achieved by the superimposition of nanoscale features onto the moderately rough surface or by alteration of the surface energy to further accelerate or increased the experimentally defined bone-to-implant contact (33-35). The possible role of surface topography in promoting more rapid and extensive bone formation at the topographically modified implant surface has been revealed in cell culture studies where molecular markers of osteoinduction and osteogenesis have repeatedly been shown to be increased at topographically enhanced titanium implant surfaces (36). The superimposition of nanoscale features on micron-rough titanium topography increases the expression of bone forming proteins beyond that observed on micron scale surfaces, suggesting that nanofeatures provide adherent osteoprogenitor cells additional cues for differentiation and subsequent bone formation (37,38). At least one human study has demonstrated that alteration of micron scale surface topography using nanofeatures increases osteoinductive gene expression during the early phases of healing (27). In a detailed investigation of adherent cell activity as a function of implant surface topography, gene expression data demonstrated that enhanced surface topography (and hydrophilicity) was associated with increase expression of genes associated with the TGFβ-BMP signaling cascade, and BMP2 protein demonstrated a large topography associated increased expression (39). Many studies have shown that surface topography is an important factor in the clinical control of osseointegration that works through the regulation of osteoinduction and subsequent bone formation.
These studies indicate that surface topography alters adherent cellular responses leading to greater bone formation. Bone accrual at the implant surface is the result of bone formation and bone resorption that occurs throughout the lifetime of the implant in function. Enhanced implant surface topography can also influence bone remodeling and osteoclast activity. This was indicated by cell culture studies of bone marrow-derived monocyte differentiation on implant surfaces cultured with osteoprogenitor cells. The rough surface provided local cues to adherent osteoprogenitors to direct osteoclast production (40,41). There are multimodal effects of enhanced surface topography that positively affect the accrual of bone at the titanium dental implant surface.
Osseointegration re-defined
Several observations made regarding the population of the implant surface by cells in vivo raised the important question of what different cells that adhere to the implant surface contribute to the process of osseointegration. In fact, the population of cells adherent to the implant surface was observed by retrieval analysis of implants in a rat model of osseointegration to change quickly over the first days and weeks of healing (42). This suggested that other cells beside osteoprogenitor or osteoblastic cells contribute to the process of osseointegration.
Recent studies have highlighted the concept of immunomodulation in the process of osseointegration. Immunomodulation refers to the modification of a biological response by cells of the immune system. In the case of osseointegration, it may be interpreted as the modification of osteoprogenitor, osteoblast and osteoclastic cells by cells of the immune system. A highly orchestrated series of cellular events must occur following tissue injury (implant surgery) to assure successful regeneration or osseointegration.
Current investigations have begun to explore how cells that populate the implant surface early and potentially prior to osteoprogenitor cells may influence osseointegration. Primary among these inflammatory cells is the macrophage. When macrophages are depleted from mice, early osseointegration is impaired, indicating that these cells do play a role in bone regeneration at dental implants (43). Macrophages polarize into so-called M1 (pro-inflammatory) and M2 (pro-regenerative) phenotypes and help direct these functions (44). Multiple investigations have demonstrated that shifting the population of macrophages to an M2 phenotype promotes the resolution of inflammation and regeneration. Endosseous implants with enhanced surface topography promote the M2 phenotype and associated enhanced osseointegration (45), while surfaces that promoted M1 phenotype impair bone regeneration. Multiple studies have now shown that macrophages play a determinant role in the process of osteoinduction that supports osseointegration. For example, in a comprehensive analysis of gene expression at titanium implant in the rat tibia, enhanced surface topography was associated with expression of osteogenesis associated gene expression that was temporally related to expression of genes associated with inflammatory/immune responses and particularly macrophages (46). In an earlier study, the expression of both osteoblast and osteoclast gene markers illustrated the aforementioned complex regulation of bone accrual at the titanium implant surface. The study did not characterize downregulated gene expression which may have included inflammation-related transcripts (47). A recent in vivo study illustrated that macrophages play a role in MSC and T-cell recruitment to titanium implant/bone interfaces (48).
Other immune cells also appear to be influenced by surface topography and impact the process of osseointegration. T lymphocytes (Th1, Th2, Treg, and Th17 cells), B lymphocytes, dendritic cells, and macrophages are each implicated in the control of bone metabolism, often involving osteoclast formation and bone resorption. However, these cells can also be involved in regulation of osteogenesis and the implant surface appears to mediate their various functions. For example, at 10 days in the rabbit tibia model, titanium implants demonstrated a consistent upregulation of a CD4-lymphocyte reaction at the implant interface (49). T lymphocyte production of IL-17 has been shown to increase cultured osteoprogenitor cells’ osteoblastic gene expression (50). Both B- and T- cells are involved in the process of bone remodeling and T-cells are enriched resources of both OPG and RANKL that regulate osteoclastogenesis and subsequent bone resorption to affect bone mass (51). The absence of T and B cells in a mouse fracture model negatively impacted bone repair in a mouse fracture model and demonstrated the relative absence of osteoblasts at the site where bone formation would otherwise occur (52).
Neutrophils are among the first extravasated cells at wound sites, including at the dental implant surface. They are actively recruited to sites of acute inflammation where the phagocytize microbe and particles. Recent studies have investigated the effects of neutrophil interactions with various dental implant surface topographies and reveal that neutrophils are responsive to surface topography and can be activated to release inflammatory cytokines. When adherent to rougher surface topography, neutrophil inflammatory cytokine expression is reduced, suggesting a favorable bone healing response (53). The contribution of cells other than osteoprogenitors to the process of interfacial bone formation highlights the importance of more broad consideration factors influencing dental implant success.
Multiple investigators have demonstrated that the culture of MSCs on titanium surfaces with enhanced surface topography reduces pro-inflammatory gene expression and increases anti-inflammatory gene expression (54). Human studies that use an implant retrieval method to question the functionality and types of cells acting in the process of osseointegration have also highlighted the importance of immunomodulation in osseointegration (40,55). Such studies have shown that genes encoding inflammatory cytokines and chemokines are expressed by implant adherent cells and that enhanced surface topography (or hydrophilicity) can suppress or reduce the pro-inflammatory cytokine/chemokine expression associated with greater bone-to-implant contact.
Osseointegration disturbed
Given the growing knowledge base regarding the complex molecular and cellular basis of osseointegration, it is possible to reconsider dental implant failure in that context. In fact, many of the aforementioned studies have shown that the use of BMPs, PTH, Wnts, inhibitors of osteoclastogenesis, or anti-inflammatory strategies all can enhance bone formation in animal models of disease including diabetes, osteoporosis and of aging. For example, PTH administration increased bone formation at titanium implants in an osteoporotic rabbit model (56) and increased early bone formation at implants in an aging rat model (57). The treatment of titanium implant surfaces with IL-4, a cytokine the promoted M2 (pro-regenerative) macrophage polarization and reduce pro-inflammatory cytokine production by adherent cells (58). Blocking the inhibitory action of sclerostin by linking sclerostin-neutralizing antibodies to titanium implant surfaces promoted greater osseointegration (32). These few examples demonstrate that the cellular and molecular events that control osseointegration are targetable factors that may be translated for clinical improvement of osseointegration (59).
Improving osseointegration at first glance may not seem terribly important given that there are many reports of high dental implant success and survival. However, the process of osseointegration is known to be disturbed by systemic (and local) factors. Several reviews have recently summarized some of the more common systemic factors that negatively influence dental implant success (60,61). In addition, the medications taken to address common chronic systemic conditions also reduce dental implant success at the level of bone accrual (62). Included were selective serotonin reuptake inhibitors, proton pump inhibitors, and bisphosphonates. These drugs have specific targets but also have indirect effects and potential off-target effects that can negatively influence osseointegration (63). Addressing osseointegration at its cellular/molecular level may provide solutions to these known clinical challenges.
Conclusions
Osseointegration has proven to be a biologically sound foundation for contemporary dental implant therapy. Its success is dependent on principle-driven clinical procedures. The formation of bone at the titanium dental implant surface is dependent on osteoprogenitor cell recruitment, proliferation and differentiation under complex control. The current relatively high success of dental implant therapy is due, in part, to the effects of enhanced surface topography on implant-adherent cell functions. Immunomodulation plays a key role in determining the bone forming process at endosseous dental implants and underscores the important relationship between the technical aspects of dental implant therapy and the local and systemic biological factors acting upon the population of implant-adherent and adjacent cells.
Acknowledgments
Funding: None.
Footnote
Provenance and Peer Review: This article was commissioned by the Guest Editor (Ole T. Jensen) for the series “Current Advances in Treatment of Peri-Implantitis” in Frontiers of Oral and Maxillofacial Medicine. The article has undergone external peer review.
Reporting Checklist: The authors have completed the Narrative Review reporting checklist. Available at https://fomm.amegroups.com/article/view/10.21037/fomm-21-77/rc
Conflicts of Interest: Both authors have completed the ICMJE uniform disclosure form (available at https://fomm.amegroups.com/article/view/10.21037/fomm-21-77/coif). The series “Current Advances in Treatment of Peri-Implantitis” was commissioned by the editorial office without any funding or sponsorship. The authors have no other conflicts of interest to declare.
Ethical Statement:
Open Access Statement: This is an Open Access article distributed in accordance with the Creative Commons Attribution-NonCommercial-NoDerivs 4.0 International License (CC BY-NC-ND 4.0), which permits the non-commercial replication and distribution of the article with the strict proviso that no changes or edits are made and the original work is properly cited (including links to both the formal publication through the relevant DOI and the license). See: https://creativecommons.org/licenses/by-nc-nd/4.0/.
References
- Bolender CL. Indications and contraindications for different types of implant therapy. J Dent Educ 1988;52:757-9. [Crossref] [PubMed]
- Brånemark PI, Hansson BO, Adell R, et al. Osseointegrated implants in the treatment of the edentulous jaw. Experience from a 10-year period. Scand J Plast Reconstr Surg Suppl 1977;16:1-132. [PubMed]
- Brånemark PI. Osseointegration and its experimental background. J Prosthet Dent 1983;50:399-410. [Crossref] [PubMed]
- Donath K, Breuner G. A method for the study of undecalcified bones and teeth with attached soft tissues. The Säge-Schliff (sawing and grinding) technique. J Oral Pathol 1982;11:318-26. [Crossref] [PubMed]
- Linder L, Albrektsson T, Brånemark PI, et al. Electron microscopic analysis of the bone-titanium interface. Acta Orthop Scand 1983;54:45-52. [Crossref] [PubMed]
- Schroeder A, Pohler O, Sutter F. Tissue reaction to an implant of a titanium hollow cylinder with a titanium surface spray layer. SSO Schweiz Monatsschr Zahnheilkd 1976;86:713-27. [PubMed]
- Albrektsson T, Brånemark PI, Hansson HA, et al. Osseointegrated titanium implants. Requirements for ensuring a long-lasting, direct bone-to-implant anchorage in man. Acta Orthop Scand 1981;52:155-70. [Crossref] [PubMed]
- Adell R. Tissue integrated prostheses in clinical dentistry. Int Dent J 1985;35:259-65. [PubMed]
- Albrektsson T, Albrektsson B. Osseointegration of bone implants. A review of an alternative mode of fixation. Acta Orthop Scand 1987;58:567-77. [Crossref] [PubMed]
- Albrektsson T, Zarb G, Worthington P, et al. The long-term efficacy of currently used dental implants: a review and proposed criteria of success. Int J Oral Maxillofac Implants 1986;1:11-25. [PubMed]
- Esposito M, Grusovin MG, Chew YS, et al. One-stage versus two-stage implant placement. A Cochrane systematic review of randomised controlled clinical trials. Eur J Oral Implantol 2009;2:91-9. [PubMed]
- Stanford CM, Keller JC, Solursh M. Bone cell expression on titanium surfaces is altered by sterilization treatments. J Dent Res 1994;73:1061-71. [Crossref] [PubMed]
- Schwartz Z, Boyan BD. Underlying mechanisms at the bone-biomaterial interface. J Cell Biochem 1994;56:340-7. [Crossref] [PubMed]
- Boyan BD, Hummert TW, Dean DD, et al. Role of material surfaces in regulating bone and cartilage cell response. Biomaterials 1996;17:137-46. [Crossref] [PubMed]
- Caplan AI. Mesenchymal stem cells. J Orthop Res 1991;9:641-50. [Crossref] [PubMed]
- Su P, Tian Y, Yang C, et al. Mesenchymal Stem Cell Migration during Bone Formation and Bone Diseases Therapy. Int J Mol Sci 2018;19:2343. [Crossref] [PubMed]
- Dimitriou R, Tsiridis E, Giannoudis PV. Current concepts of molecular aspects of bone healing. Injury 2005;36:1392-404. [Crossref] [PubMed]
- Davies JE. Understanding peri-implant endosseous healing. J Dent Educ 2003;67:932-49. [Crossref] [PubMed]
- Olsen BR, Reginato AM, Wang W. Bone development. Annu Rev Cell Dev Biol 2000;16:191-220. [Crossref] [PubMed]
- Souza JCM, Sordi MB, Kanazawa M, et al. Nano-scale modification of titanium implant surfaces to enhance osseointegration. Acta Biomater 2019;94:112-31. [Crossref] [PubMed]
- Boyan BD, Cheng A, Olivares-Navarrete R, et al. Implant Surface Design Regulates Mesenchymal Stem Cell Differentiation and Maturation. Adv Dent Res 2016;28:10-7. [Crossref] [PubMed]
- Ducy P, Zhang R, Geoffroy V, et al. Osf2/Cbfa1: a transcriptional activator of osteoblast differentiation. Cell 1997;89:747-54. [Crossref] [PubMed]
- Komori T, Yagi H, Nomura S, et al. Targeted disruption of Cbfa1 results in a complete lack of bone formation owing to maturational arrest of osteoblasts. Cell 1997;89:755-64. [Crossref] [PubMed]
- Chatakun P, Núñez-Toldrà R, Díaz López EJ, et al. The effect of five proteins on stem cells used for osteoblast differentiation and proliferation: a current review of the literature. Cell Mol Life Sci 2014;71:113-42. [Crossref] [PubMed]
- Masaki C, Schneider GB, Zaharias R, et al. Effects of implant surface microtopography on osteoblast gene expression. Clin Oral Implants Res 2005;16:650-6. [Crossref] [PubMed]
- Guo J, Padilla RJ, Ambrose W, et al. The effect of hydrofluoric acid treatment of TiO2 grit blasted titanium implants on adherent osteoblast gene expression in vitro and in vivo. Biomaterials 2007;28:5418-25. [Crossref] [PubMed]
- Bryington M, Mendonça G, Nares S, et al. Osteoblastic and cytokine gene expression of implant-adherent cells in humans. Clin Oral Implants Res 2014;25:52-8. [Crossref] [PubMed]
- Donos N, Hamlet S, Lang NP, et al. Gene expression profile of osseointegration of a hydrophilic compared with a hydrophobic microrough implant surface. Clin Oral Implants Res 2011;22:365-72. [Crossref] [PubMed]
- Li Z, Yuan X, Arioka M, et al. Pro-osteogenic Effects of WNT in a Mouse Model of Bone Formation Around Femoral Implants. Calcif Tissue Int 2021;108:240-51. [Crossref] [PubMed]
- Boyan BD, Olivares-Navarrete R, Berger MB, et al. Role of Wnt11 during Osteogenic Differentiation of Human Mesenchymal Stem Cells on Microstructured Titanium Surfaces. Sci Rep 2018;8:8588. [Crossref] [PubMed]
- da Silva FL, Alves Maced CA, Peruzzo DC, et al. Preliminary Findings on the Role of Sclerostin in the Osseointegration Process Around Titanium Implants. Int J Oral Maxillofac Implants 2016;31:1298-302. [Crossref] [PubMed]
- Yu SH, Hao J, Fretwurst T, et al. Sclerostin-Neutralizing Antibody Enhances Bone Regeneration Around Oral Implants. Tissue Eng Part A 2018;24:1672-9. [Crossref] [PubMed]
- El Chaar E, Zhang L, Zhou Y, et al. Osseointegration of Superhydrophilic Implants Placed in Defect Grafted Bones. Int J Oral Maxillofac Implants 2019;34:443-450. [Crossref] [PubMed]
- Berglundh T, Abrahamsson I, Albouy JP, et al. Bone healing at implants with a fluoride-modified surface: an experimental study in dogs. Clin Oral Implants Res 2007;18:147-52. [Crossref] [PubMed]
- Abrahamsson I, Albouy JP, Berglundh T. Healing at fluoride-modified implants placed in wide marginal defects: an experimental study in dogs. Clin Oral Implants Res 2008;19:153-9. [Crossref] [PubMed]
- Wall I, Donos N, Carlqvist K, et al. Modified titanium surfaces promote accelerated osteogenic differentiation of mesenchymal stromal cells in vitro. Bone 2009;45:17-26. [Crossref] [PubMed]
- Gittens RA, McLachlan T, Olivares-Navarrete R, et al. The effects of combined micron-/submicron-scale surface roughness and nanoscale features on cell proliferation and differentiation. Biomaterials 2011;32:3395-403. [Crossref] [PubMed]
- Mendonça G, Mendonça DB, Aragão FJ, et al. The combination of micron and nanotopography by H(2)SO(4)/H(2)O(2) treatment and its effects on osteoblast-specific gene expression of hMSCs. J Biomed Mater Res A 2010;94:169-79. [Crossref] [PubMed]
- Vlacic-Zischke J, Hamlet SM, Friis T, et al. The influence of surface microroughness and hydrophilicity of titanium on the up-regulation of TGFβ/BMP signalling in osteoblasts. Biomaterials 2011;32:665-71. [Crossref] [PubMed]
- Nagasawa M, Cooper LF, Ogino Y, et al. Topography Influences Adherent Cell Regulation of Osteoclastogenesis. J Dent Res 2016;95:319-26. [Crossref] [PubMed]
- Yang J, Yu X, Zhang Z, et al. Surface modification of titanium manufactured through selective laser melting inhibited osteoclast differentiation through mitogen-activated protein kinase signaling pathway. J Biomater Appl 2020;35:169-81. [Crossref] [PubMed]
- Masuda T, Salvi GE, Offenbacher S, et al. Cell and matrix reactions at titanium implants in surgically prepared rat tibiae. Int J Oral Maxillofac Implants 1997;12:472-85. [PubMed]
- Wang X, Li Y, Feng Y, et al. The role of macrophages in osseointegration of dental implants: An experimental study in vivo. J Biomed Mater Res A 2020;108:2206-16. [Crossref] [PubMed]
- Loi F, Córdova LA, Zhang R, et al. The effects of immunomodulation by macrophage subsets on osteogenesis in vitro. Stem Cell Res Ther 2016;7:15. [Crossref] [PubMed]
- Ma QL, Zhao LZ, Liu RR, et al. Improved implant osseointegration of a nanostructured titanium surface via mediation of macrophage polarization. Biomaterials 2014;35:9853-67. [Crossref] [PubMed]
- Thalji G, Gretzer C, Cooper LF. Comparative molecular assessment of early osseointegration in implant-adherent cells. Bone 2013;52:444-53. [Crossref] [PubMed]
- Kojima N, Ozawa S, Miyata Y, et al. High-throughput gene expression analysis in bone healing around titanium implants by DNA microarray. Clin Oral Implants Res 2008;19:173-81. [Crossref] [PubMed]
- Abaricia JO, Shah AH, Chaubal M, et al. Wnt signaling modulates macrophage polarization and is regulated by biomaterial surface properties. Biomaterials 2020;243:119920. [Crossref] [PubMed]
- Trindade R, Albrektsson T, Galli S, et al. Bone Immune Response to Materials, Part I: Titanium, PEEK and Copper in Comparison to Sham at 10 Days in Rabbit Tibia. J Clin Med 2018;7:526. [Crossref] [PubMed]
- Croes M, Öner FC, van Neerven D, et al. Proinflammatory T cells and IL-17 stimulate osteoblast differentiation. Bone 2016;84:262-70. [Crossref] [PubMed]
- Könnecke I, Serra A, El Khassawna T, et al. T and B cells participate in bone repair by infiltrating the fracture callus in a two-wave fashion. Bone 2014;64:155-65. [Crossref] [PubMed]
- El Khassawna T, Serra A, Bucher CH, et al. T Lymphocytes Influence the Mineralization Process of Bone. Front Immunol 2017;8:562. [Crossref] [PubMed]
- Abaricia JO, Shah AH, Musselman RM, et al. Hydrophilic titanium surfaces reduce neutrophil inflammatory response and NETosis. Biomater Sci 2020;8:2289-99. [Crossref] [PubMed]
- Hyzy SL, Olivares-Navarrete R, Hutton DL, et al. Microstructured titanium regulates interleukin production by osteoblasts, an effect modulated by exogenous BMP-2. Acta Biomater 2013;9:5821-9. [Crossref] [PubMed]
- Ivanovski S, Hamlet S, Salvi GE, et al. Transcriptional profiling of osseointegration in humans. Clin Oral Implants Res 2011;22:373-81. [Crossref] [PubMed]
- Almagro MI, Roman-Blas JA, Bellido M, et al. PTH 1-34 enhances bone response around titanium implants in a rabbit model of osteoporosis. Clin Oral Implants Res 2013;24:1027-34. [PubMed]
- Jiang L, Zhang W, Wei L, et al. Early effects of parathyroid hormone on vascularized bone regeneration and implant osseointegration in aged rats. Biomaterials 2018;179:15-28. [Crossref] [PubMed]
- Wang Y, Qi H, Miron RJ, et al. Modulating macrophage polarization on titanium implant surface by poly(dopamine)-assisted immobilization of IL4. Clin Implant Dent Relat Res 2019;21:977-86. [Crossref] [PubMed]
- Alenezi A, Chrcanovic B, Wennerberg A. Effects of Local Drug and Chemical Compound Delivery on Bone Regeneration Around Dental Implants in Animal Models: A Systematic Review and Meta-Analysis. Int J Oral Maxillofac Implants 2018;33:e1-e18. [Crossref] [PubMed]
- Chrcanovic BR, Kisch J, Albrektsson T, et al. Analysis of risk factors for cluster behavior of dental implant failures. Clin Implant Dent Relat Res 2017;19:632-42. [Crossref] [PubMed]
- Chrcanovic BR, Kisch J, Albrektsson T, et al. Factors Influencing Early Dental Implant Failures. J Dent Res 2016;95:995-1002. [Crossref] [PubMed]
- Aghaloo T, Pi-Anfruns J, Moshaverinia A, et al. The Effects of Systemic Diseases and Medications on Implant Osseointegration: A Systematic Review. Int J Oral Maxillofac Implants 2019;34:s35-49. [Crossref] [PubMed]
- Chappuis V, Avila-Ortiz G, Araújo MG, et al. Medication-related dental implant failure: Systematic review and meta-analysis. Clin Oral Implants Res 2018;29:55-68. [Crossref] [PubMed]
Cite this article as: Cooper LF, Shirazi S. Osseointegration—the biological reality of successful dental implant therapy: a narrative review. Front Oral Maxillofac Med 2022;4:39.